Impact of the Proposed Introduction of 3 MHz and 5 MHz Bandwidths to the Non-licensed 2.4 MHz Band
This is the first in a series of bi-monthly columns from Philsar engineers on Bluetooth related issues. The column will be published in the middle of every-other-month, starting with this month's installment.
Contents
Frequency of collision
BER and compression
On-channel spectral densities
Adjacent channel interference
Consequences and corrective measures
In recent years the Federal Communications Commission (FCC) has permitted the operation of non-licensed radio frequency devices in the 2.4 GHz band, at higher power levels than are permissible at other frequency bands, based on a requirement for the use of spread spectrum signaling. The use of spread spectrum signaling not only minimizes the interference to other authorized services; it also minimizes the interference from such services to itself. As a result, great interest and activity has culminated in industry-led standardization, such as Bluetooth.
Under the existing regulations, a frequency hopping spread spectrum system operating in the 2.4 GHz band must use at least 75 hopping frequencies, with a 20 dB hopping channel bandwidth of 1 MHz. Further, the maximum radiated power must not exceed 1 W, and the average time of occupancy on any frequency must not exceed 400 msec. within a 30-second period.
In order to be facilitating high-speed data links for applications such as wireless LANs, the FCC is considering increases to 3 MHz and 5 MHz bandwidths. However the impact of more frequent collisions (due to the wider bandwidths and faster hopping rates) and higher interfering power-spectral-densities on the present 1 MHz systems must be considered.
While the business opportunity of increased channel bandwidths is clearly evident, the compatibility of such bandwidths with the present 1 MHz bandwidth systems requires further investigation. In addition to the increased bandwidths, the proposed FCC rule change includes tighter restrictions on the radiated power and average time of occupancy. A summary is given in Table 1.
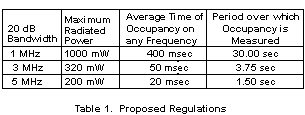
Frequency of collision
The frequency of collisions between a 3 or 5 MHz signal and a 1 MHz signal is a concern. From Table 1, we see that the percentage time occupancy of a 3 or 5 MHz signal on a specific channel remains the same as that for the 1 MHz signal. For example, for the 5 MHz signal, 20 msec. occupancy / 1.5 sec. period remains 0.0133, the same as for the 1 MHz signal.
However, the true measure of frequency of collision is obtained from the relative bandwidths and the relative hop duration of the 3 or 5 MHz signal as compared to the 1 MHz signal. Since the new bandwidth signals occupy three or five times the bandwidth of the 1 MHz signal, they will have three or five times the probability of colliding with (a portion of the spectrum of) the 1 MHz signal. Further, if the new bandwidth signals employ faster hop rates, then they will have a higher probability of colliding with (a portion of the duration of) a 1 MHz signal hop.
For example, if a new 3 MHz bandwidth signal hops at three times the rate of a 1 MHz signal, then there is 3 x 3 = 9 times the opportunity to collide at least once with a portion of an individual hop of the 1 MHz signal.
BER and compression
In addition to affecting bit-error-rate performance, the 3 and 5 MHz signals can also cause compression of the desired receiver through IP2 mechanisms, resulting in a DC-compensation requirement. With the increase in the frequency of collisions during an individual hop, some Bluetooth solutions may not be able to maintain their averaged BER performance, while other solutions may not be able to cope with the increased demand for DC compensation.
On-channel spectral densities
From Table 1 it can be seen that, if a (on-channel) collision does occur between a 3 or 5 MHz signal and a 1 MHz signal, the effect of that collision on the 1 MHz signal will be less than that of a collision with another 1 MHz signal. This is due to the decrease in maximum radiated power of the 3 or 5 MHz signal, and to the reduction in power spectral density resulting from the increased bandwidth.
For example, if a collision with a 5 MHz signal occurs, its interfering power is 1/5th that of another 1 MHz signal. Further only 1/5th of its interfering power exists in the 1 MHz band of the 1 MHz signal. Hence its interference effect is 1/25th that of another 1 MHz signal, assuming the same distances and propagation effects.
Table 2 compares the reduction in interference effect of the 3 MHz and 5 MHz signals as compared to another 1 MHz signal.
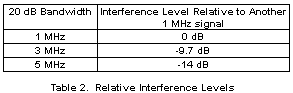
Adjacent channel interference
For adjacent channel signals, the width and shape of the power-spectral-density of the 3 or 5 MHz signal must be considered with respect to that for a 1MHz signal as shown in Figure 1. A 5 MHz signal will have significantly more power in the adjacent band than will a 1 MHz signal. Simulations have shown that to maintain the same bit error rate performance, a 3 or 5 MHz signal should intersect with the desired signal at virtually the same power spectral density value as occurs with a 1 MHz signal. This translates into a power reduction requirement of typically 12 dB for a 3 MHz signal in the adjacent channel, and of typically 17 dB for a 5 MHz signal in the adjacent channel as compared with a 1 MHz signal.
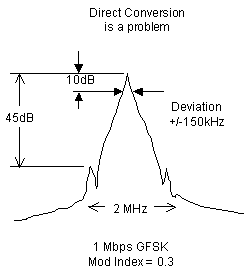
Consequences and corrective measures
The proposed reductions in power levels for the 3 MHz and 5 MHz signals result in adjacent channel interference levels that fall short of what is required by typically 2.5 to 3.0 dB. This, combined with the increase in collision rates, may render some Bluetooth solutions inoperable.
If the proposed FCC Notice of Proposed Rule Making is adopted, care must be taken to counter such collisions as best as possible. Linear receivers having high compression points and good filter roll-offs could be incorporated into the RF circuitry, all-be-it with higher DC current draw. Also techniques such as symbol interleaving and symbol repetition, combined with convolutional coding could be used.
About the author:
Jim Wight is a Senior Radio Architect for Conexant Systems Inc. In addition to his work with Conexant, Wight is a professor of engineering at Carleton University, Ottawa, Ontario Canada.